Access Laser was started when Dr. Zhang saw CO2 laser companies entrenching further towards the dollar-per-watt paradigm and in the process ignoring the scientific and research markets. This led to the focus and core customer base generated during the first fifteen years of the company’s existence. By providing high quality and robust light sources for instrumentation and measurement applications Access Laser built its reputation as the one-stop-shop of precision CO2 lasers. The ability to combine high power stability with the full CO2 spectral range of 9-11µm in our -G models across our various product offerings made an attractive statement to laser researchers. However, in recent years Quantum Cascade Lasers (QCL) have been improving their performance and lowering their prices, providing an alternative for some researchers where acceptable.
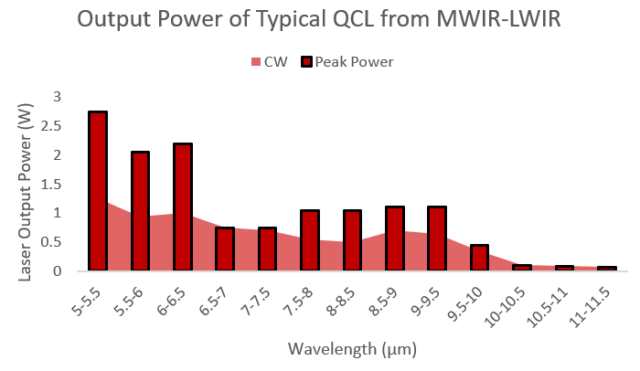
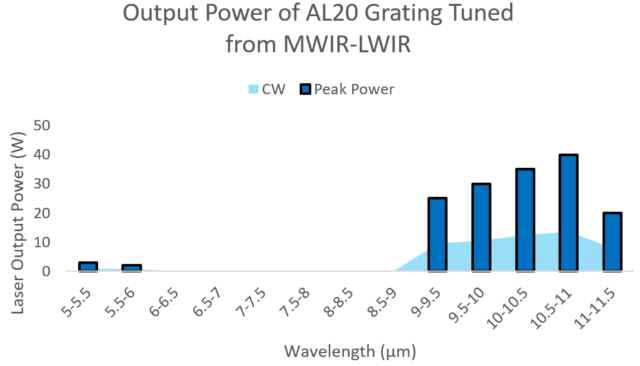
Figure 1: Output power vs wavelength from a commercial QCL & Access Laser AL20-G-WC
While QCL’s do provide excellent performance in specific applications, there are fundamental limitations where CO2 lasers remain the ideal source. Spatial mode quality, spectral purity and of course output power all remain key advantages for high-performance CO2 lasers which cannot be matched by competing sources. These optical features play crucial roles in interferometry, spectroscopy, standoff detection and precision heating for manipulation of optical fibers, as well as custom freeform optics.
In spectroscopy there are many techniques which can leverage the power of our CO2 lasers that exceeds the milliwatt range of QCL sources. Cavity RingDown Spectroscopy (CRDS) is one such example which uses our AL50-GS-WC for measuring the differential absorption of volitile organic compounds (e.g. ethane, n-pentane, ethanol, acetone) in breath samples for early detection of lung cancer and several other deseases.
Another area of spectroscopy where our CO2 lasers maintain a strong advantage is for photoacoustic investigation. Here, you can distinctly define the multi-wave fingerprints of molecules such as Ethylene or Ammonia. This is done in a five-step process:
- Modulation of the laser radiation at a wavelength which overlaps with the spectral features of the target species.
- Excitation of a fraction of the ground-state population of the target molecule by absorption of the laser radiation in a vibrational-rotational energy level.
- Energy exchange between these vibrational levels and from vibrational to rotational and translational degrees of freedom. This is almost completely converted to kinetic energy of the gas molecules by collisional de-excitation
- Expansion and contraction of the gas in a closed volume from these collisions gives rise to pressure variations which can be measured as acoustic waves
- Monitoring the resultant wave provides insight to the gas species, concentration and other characteristics useful to researchers.
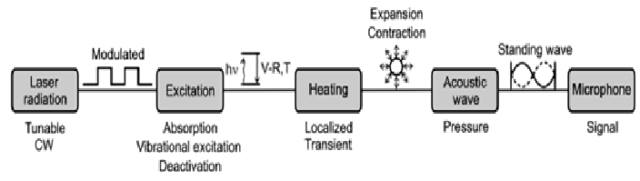
Figure 2: Schematic of the physical processes occurring during optical excitation of molecules in photoacoustic spectroscopy1
Finally, you can review the below comparison table between leading QCL performance and an Access Laser CO2 source:
ALC SLE | QCL | |
Wavelength Range (µm) | 9-11.2 | 3-12 |
Within Range Coverage | discrete | Continuous |
Frequency accuracy (cm-1) | 0.01 | 1 |
Frequency stability (cm-1) | 0.01 | 1 |
Line width (MHz) | 0.1 | 100 |
Max Average Power (W) | 50 | 0.5 |
Max peak power (W) | 1000 | 1 |
Efficiency (DC to light) | 3% | <1% |
Specific volume (in3/W) | 135 | 2400 |
Upper modulation frequency | 5kHz Direct, 100kHz Q-switch | Up to 1 MHz |
Modulation frequency with external modulators | >100 MHz | >100 MHz |
Water cooling | Not required for some models | Yes for full operation |
References:
- C. Lindon, G. E. Tranter, J. L. Holmes,” Encyclopedia of Spectroscopy and Spectrometry”, Academic Press, 2010.